Research
We develop unconventional biochemical sensors and energy devices for applications in wearables, robotics, and neuroscience. We build upon foundational scientific theories and engineering advances in the field of self-powered sensors, energy harvesters, bioelectronic composites, heterogeneous fabrication, and structure-property relations to develop self-powered/low-powered tissue-integrated devices. Devices for such applications are crucial for obtaining deep insights into the functioning of the human body and for developing advanced robotics, yet this field remains understudied due to dependence on energy intensive sensing approaches, challenges in device fabrication, lack of robust sensing biomaterials, and insufficient collaboration between engineering, science, and medicine. The group’s unparalleled expertise in biochemical sensors, energy harvesters, soft electronics, and unusual fabrication techniques and collaborations with physicists, physicians, neuroscientists, and biologists uniquely positions the group within the scientific community to develop the next generation of conformal, self-powered/low-power biochemical sensors and energy devices with broad applications in wearables, robotics, and implantables.
Next-generation wearable sensors
Wearable biochemical sensors hold vast potential for revolutionizing personalized medicine and advanced robotics, yet present research narrowly focuses on metabolites and electrolytes primarily relevant to athletics and fitness. Dependence on energy intensive sensing principles, lack of robust sensing materials, and challenges in fabrication processes are major limitations facing the field of wearable biochemical sensors. We seek to address these issues and expand the gamut of application for wearable sensors to areas beyond general fitness, such as, disease monitoring and advanced robotics by building upon our expertise in unconventional biochemical sensing techniques, electronic materials processing, advanced microfluidics, and systems engineering.
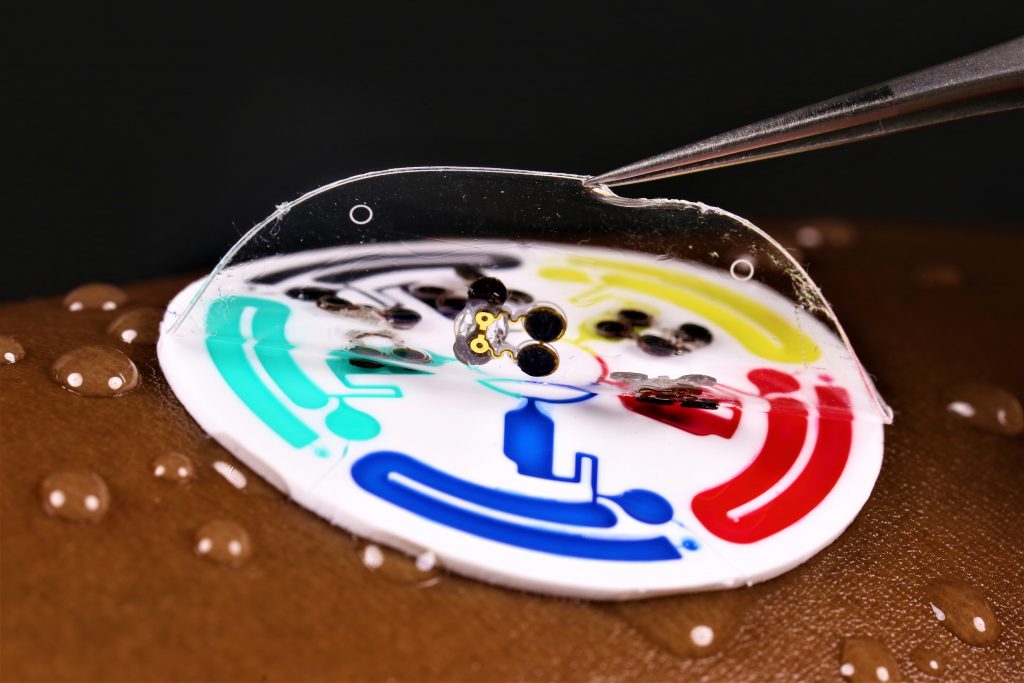
Advanced neurochemical sensors
Comprehensive understanding of neural circuitry requires region-specific mapping of neurochemical activity in live animals. The conventional method of micro-dialysis is complicated, doesn’t permit true real-time analysis, and causes severe stress to animals. Present approaches that include electrochemical sensors can detect a handful of neurochemicals while fluorescent probes face limitations like poor photostability and the need for multiple separate optical probes to excite and capture signals. We aim to exploit our expertise in bioelectrochemistry, materials processing, fabrication processes, and heterogeneous assembly to overcome these issues and develop wireless neural probes for monitoring a wide range of previously undetectable neurochemicals in freely moving animals.
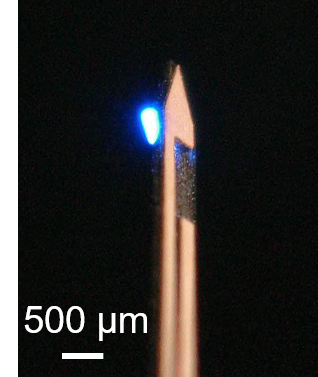
Unusual power sources
Power sources are a vital component of a wide range of tissue-integrated devices. However mainstream alternatives, such as, commercial batteries and supercapacitors not designed for such unique bio-applications. Their rigid and bulky form-factor and dependence on toxic chemicals diminish their attractiveness for wearable and implantable applications. While recent demonstrations of conformal, non-toxic power sources overcome some of the issues, these systems still face problems associated with short shelf-life and poor integration. We pursue to address these challenges by exploiting our experience in energy materials, electrochemistry, heterogenous fabrication, and integrated systems to develop new classes of non-traditional power sources in the form of biofuel cells and biocompatible batteries for advanced biomedical applications.
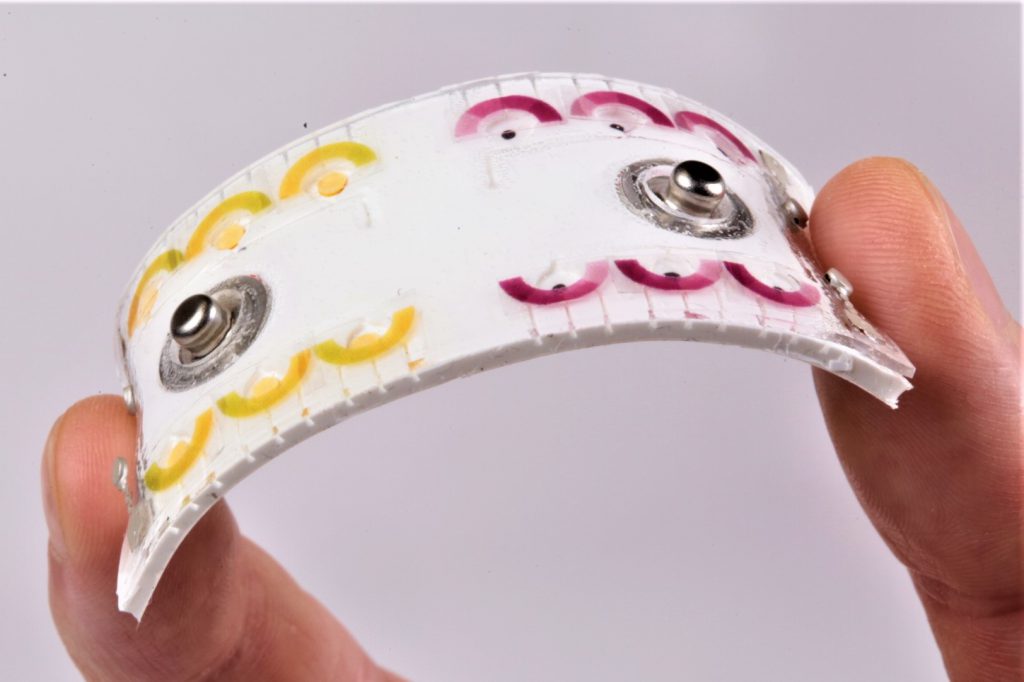
Hybrid fabrication processes
Lithography and printing are the predominant fabrication processes used for developing soft, stretchable sensors and energy devices for various bio-integrated applications. While lithography results in stretchable devices with performance similar to conventional systems, the narrow range of compatible materials is a major drawback. Alternatively, a rich variety of functional materials that are essential for sensors and energy devices can be processed via printing. Unfortunately, broadly used methods of developing stretchable printed systems require incorporation of considerable amounts of nonconductive elastomeric binders that lead to devices with sub-optimal performance. We wish to address these fabrication challenges by exploring a synergistic approach that leverages attributes of new classes of smart inks, lithography and laser patterning for largescale production of high-performance stretchable sensors and energy systems.
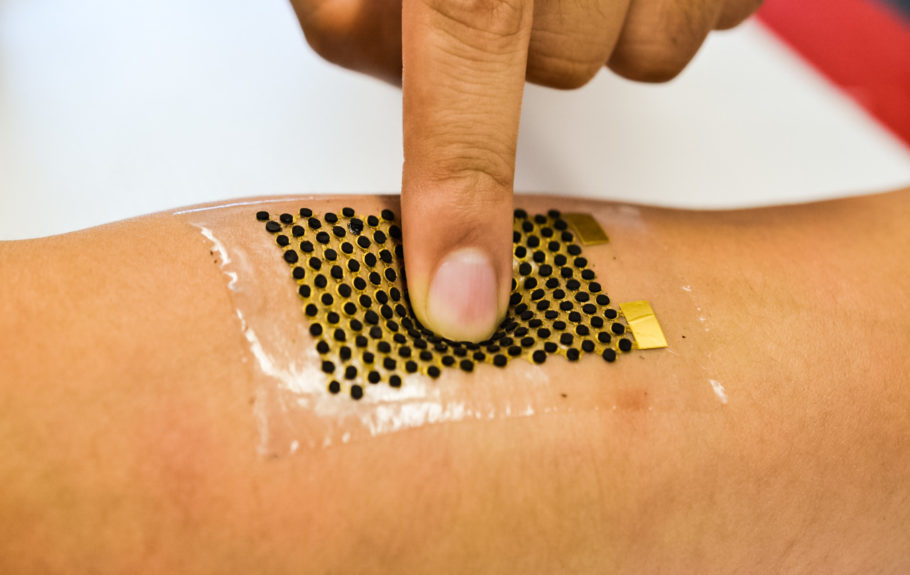